A neutron star is the result of a star that starts with a mass between approximately 8 and 20 times the mass of the sun, and experiences a supernova. Having formed, it is about 15 to 30 km (10 to 20 miles) in diameter with a mass approximately 1.4 to 2 times that of the Sun. On Earth, one teaspoonful would weigh a billion tons. An often-quoted comparison is that the core density of a Neutron Star is the same as condensing the entire population of the Earth to the size of a sugar cube. The average density is comparable to the density of an atomic nucleus. Click here to see a picture that represents the current understanding of the interior of a Neutron Star.
So, how does a neutron star form? We discussed the basics under high mass stars, above. Having exhausted energy production from fusion, the core collapses. No radiation flows from the core, so it cannot support the middle and upper layers against the force of gravity. The pressure of the in-falling material squeezes the electrons and protons of the core together to create neutrons and neutrinos. The neutrinos fly into space, taking some energy with them, while the neutrons are forced together until they reach the density of an atomic nucleus.
The middle layers bounce off the solid neutron core, and the shock wave blows off the upper layers as a Supernova leaving behind a beautiful nebula like the one in the Crab Nebula, see picture below. That Supernova was seen on Earth in 1054. Because of the conservation of most of the angular momentum of the original star, the Neutron Star usually spins rapidly. The rate of rotation is, generally, between 1.4 milliseconds (so-called milli-second Pulsars) and 30 seconds. The strong magnetic field produces dual narrow beams of radio waves from the magnetic poles. When the magnetic poles are offset from the axis of rotation, the beams swing around like the light from a lighthouse. From Earth, it appears that the star is flashing on and off as it spins; this type of Neutron Star, where we can see the rotating beams, is called a Pulsar.
Another type of Neutron star is a magnetar. They are characterized by enormous magnetic fields; perhaps 1,000 times as strong as a normal neutron star, and the strongest magnetic field known in the Universe. As the magnetic field decays, it powers the emission of very high-energy electromagnetic radiation, particularly X-rays and gamma rays. As a star collapses to a neutron star, its magnetic field strength increases by a factor of four for every halving of the star's diameter. A star reduces in diameter to about 20 miles while becoming a neutron star, so the increase in magnetic strength is huge. The decay process is such that after about 10,000 years, there is no longer enough magnetism to continue powering the strong electromagnetic emission. Soft gamma repeaters and anomalous X-ray pulsars are widely considered to be magnetars. Some astronomers suggest that the only way the magnetic field of a magnetar could become so strong is if it was actually a (hypothetical) quark star, but this is highly conjectural.
PSR B1919+21
No discussion of Neutron Stars or Pulsars can omit PSR B1919+21, or LGM1 as it was called on its discovery - "Little Green Men 1". Discovered in 1967 by Jocelyn Bell and Antony Hewish, its incredibly accurate radio pulsations lead to speculation that it could be a communication from intelligent extraterrestrials. However, Thomas Gold and Fred Hoyle identified the signals as emanating from a rapidly rotating, highly magnetic neutron star, subsequently identified as CP1919, and now known as PSR B1919+21. It pulsates every 1.3373 seconds, is about 20 km (12.5 miles) in diameter, weighs about 1.4 solar masses, and is 2,283.12 light-years away. The neutron star formed about 16 million years ago.
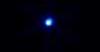
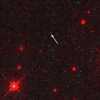
Neutron Stars
The Crab Nebula is the remnant of a supernova that occured in 1054, which was recorded by Chinese and Arab astronomers at the time. It is about 6,500 light-years away, and, after less than 1,000 years, is already about 11 ly across as it is expanding at about 1,500 km (nearly 1,000 miles) per second. The Pulsar, or rotating Neutron star, which is the driving force behind the nebula, is about 25 to 30 km (~ 15 to 19 miles) in diameter, and pulses every 33 milliseconds. While the pulses are incredibly precisely timed, like all pulsars, the Crab pulsar is slowing down in a very predictable way. In the Crab's case, this is by 38 nanoseconds per day, and is due to the large amounts of energy being carried away in the pulsar wind which is what drives the gas and dust out. The three images show the whole nebula, the central portion highlighting the neutron star, and a combined optical (hubble) & X-ray (chandra) image.
PSR B0531+21 (The Crab Pulsar)
RX J185635-3754
This is the star RX J185635-3754, the first Neutron Star ever observed in visible light. It is one of the closest known neutron stars, estimated to be about 400 light-years away (note: earlier estimates of 150 to 200 light-years away were incorrect). It has a surface temperature of approximately 434,000ºK, and it radiates mainly in X-rays. Combined with the small diameter of a Neutron Star, it is very dim. Data from the Chandra and Hubble telescopes now lead to a modified estimated diameter of some 14 km (8.75 miles), which is rather larger than earlier estimates. Thus the star is no longer considered to be a quark star candidate.
PSR B1509-58 is a pulsar that is spinning nearly seven times a second, while pouring huge quantities of energy into space due to its enormous magnetic field estimated to be 18 x 1012 times (18 trillion) stronger than the earth's. However, see SGR 1806-20, below, for really strong magnetic fields. The neutron star is thought to have formed from a supernova that occurred about 1,700 years ago, and is about 170,000 light years away. It has produced the stunning nebula shown to the right which is about 150 light years long. The photograph is from Chandra, and blue indicates the highest energy X-Rays with green being intermediate and red the lowest energy.
PSR B1509-58
No picture, but the recently discovered neutron star J1614-2230 is the heaviest identified to date. At about two solar masses, it is close to the theoretical limit on how heavy a neutron star can get without collapsing further into a black hole. The previous record holder, J1903+0327, weighed in at around 1.74 solar masses. J1614-2230 is spinning at a rate of 317 revolutions per second, and is in mutual orbit around a white dwarf star. The orbital plane of the system is almost exactly edge on to us giving astronomers a very interesting view. When the dwarf is in front of the neutron star, its pulses are delayed giving a lot of information about the make up of the system.
J1614-2230
SGR 1806-20
SGR 1806-20 is a magnetar, or SGR (soft gamma repeater) that is, thankfully, about 50,000 light-years from Earth. It has the strongest magnetic field of any known object, equivalent to about 1015 (one quadrillion) times stronger than that of the Earth. The radiation produced in one tenth of a second by an explosion on the surface of the star in 2004 was more than that produced by the Sun in 100,000 years. Despite being so distant, had the gamma radiation been in visible light, it would have been brighter than the full moon. SGR 0501+4516, another SGR, is closer at about 15,000 light years but its magnetic field is only about one tenth the strength. There are some really scary objects out there.
SGR 1900+14
SGR 1900+14 is another magnetar, or SGR, about 45,000 light years away. This picture from the Spitzer infrared telescope shows a ring that is about 7 light years across. SGR 1900+14 is in the middle, but is not actually visible in this infrared image. The bright glow at the center is a group of bright stars in the vicinity of the magnetar. The nature of the ring is unknown.
Image Credit: NASA/CXC/CfA/P. Slane et al.
Astronomy & Cosmology -
Stars - Life & Death of Stars